Title
Advances and Challenges in Graphene-Based Biosensors for Health Monitoring: A Review on Implantation, Biocompatibility, and Detoxification Solutions
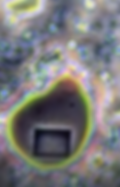
Graphenated Pegulated Ethylene Glycol(PEG) Hydrogel containing a Smart Biochip found in human blood for receiving and transmitting molecular and cellular medical data and electromagnetic treatments.. The design is an advanced biochip of graphene and ferric oxide, embedded sensors, microprocessors, and wireless communication elements, all optimized for integration within biological tissues. This micrograph above was observed in the live blood of a COVID vaccinated male emphasizes the innovative technology used in biochips being transfection into the human body fluids. Copyright Hikari Omni Media and Dr. Robert O. Young. 2024 - https://rumble.com/v3yn4bw-smart-dust-or-biotechnology-found-in-human-blood-have-you-been-biochipped.html
Author
Robert Oldham Young CPT, MSc, DSc, Phd, Naturopathic Practitioner
Keywords
Graphene field-effect transistors, G-FETs, biosensors, biocompatibility, health monitoring, Bluetooth Low Energy (BLE), encapsulation, neurochemical monitoring, continuous glucose monitoring, implantable biosensors, detoxification, MasterPeace Zeolite Z, SOLergy Sea Minerals, ethical concerns, cytotoxicity, genotoxicity.
Abstract
This review explores the development and challenges associated with graphene-based field-effect transistor (G-FET) biosensors for biomedical applications. Although promising for real-time, in-body monitoring, G-FETs face substantial challenges in biocompatibility, signal stability, and longevity that hinder their potential as implantable devices. Topics include biocompatibility modifications, encapsulation strategies, power solutions for real-time data transmission, and applications in glucose and neurochemical monitoring. Furthermore, this review addresses ethical concerns and potential cytotoxic, genotoxic, and magneto toxic effects associated with G-FET biosensors. The role of MasterPeace™ Zeolite Z™ in an aqueous colloidal solution of SOLergy™ Sea Minerals™, as a detoxifying agent for harmful components is also discussed. This article provides insights into current research, challenges, and future directions for implantable G-FET biosensors.
Introduction
Graphene-based biosensors, specifically graphene field-effect transistors (G-FETs), have emerged as promising tools for real-time health monitoring due to their high sensitivity, flexibility, and electrical conductivity [1]. Recent advancements in G-FET technology have enabled rapid, sensitive, and label-free detection of various biological molecules, proving useful for disease diagnosis [2]. However, implanting G-FET biosensors in humans presents significant challenges, particularly regarding biocompatibility, signal stability, and device durability. To overcome these barriers, recent research has focused on surface modification techniques, reliable power sources, and encapsulation strategies [3].
Review of "Graphene Field Effect Transistors for Biomedical Applications
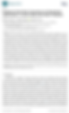
Current Status and Future Prospects, "A recent article titled "Graphene Field Effect Transistors for Biomedical Applications: Current Status and Future Prospects" reviews advancements in G-FETs, examining their potential in healthcare diagnostics and related challenges.
The following are key insights from the article:
Purpose and Significance:
G-FETs are noted for their high sensitivity, scalability, and cost-effectiveness, making them suitable for biomarker detection across various diseases [4].
Key Features and Mechanism:
Graphene’s high surface area, rapid electron mobility, and conductivity enable molecular-level detection by translating bioreceptor-target molecule interactions into electrical signals. Biofunctionalization techniques are essential for specificity and sensitivity in diagnostic use [5].
Applications in Biosensing
This article categorizes G-FET biosensors based on targeted molecules:
Nucleic Acid Sensors for detecting DNA, RNA, and microRNAs, crucial in diagnosing genetic disorders and cancers [6].
Immunosensors for identifying disease-associated protein biomarkers, such as those linked to heart failure and cancer [7].
Challenges and Future Directions
Limitations include Debye-Hückel screening in biological samples and restricted graphene surface area for biomolecule interactions. Improved biofunctionalization and nanoparticle-enhanced surfaces are areas of active research to address these challenges [8].
Implantation and Biocompatibility Challenges
Cytotoxicity and Genotoxicity Risks: Implantable G-FET biosensors pose potential risks of cytotoxicity and genotoxicity. Graphene's nanoscale features enable close cellular interaction, which may damage cell membranes and DNA, respectively, potentially leading to inflammation, mutations, and even cancer development over time [9].
Immunogenicity and Encapsulation Needs: The body's immune response to graphene-based devices requires protective encapsulation materials, like parylene or silicone, to mitigate inflammatory responses and ensure stability in biological environments. Biocompatible coatings such as PEG and dextran reduce biofouling and enhance device longevity [10][11].
Magneto Toxicity Concerns: When paired with magnetic nanoparticles, graphene-based devices might interact adversely with magnetic fields, presenting risks in clinical environments where patients undergo MRI scans. This interaction could destabilize or impair device function, highlighting a need for careful design [12].
Power and Communication Needs: Wireless power transfer and Bluetooth Low Energy (BLE) systems are being explored to power G-FET biosensors and facilitate real-time data communication without wires, a critical advancement for continuous monitoring [13].
Applications and Research Progress
Continuous Glucose Monitoring: G-FET biosensors offer promise for minimally invasive, continuous glucose monitoring, particularly for diabetic patients. These sensors can potentially transmit data wirelessly, reducing the need for frequent blood samples [14].
Neurochemical Monitoring: G-FETs are under investigation for monitoring neurochemicals like dopamine and serotonin, which could benefit individuals with neurological conditions. These applications remain experimental, focusing on achieving stable implantation and precise monitoring [15].
Ethical and Health Concerns Regarding Implantable Graphene-Based Biosensors
The application of graphene-based biosensors for health monitoring raises significant ethical and health concerns, particularly when considering the implantation of foreign materials in humans or animals. While G-FET biosensors hold great potential, they also pose risks such as cytotoxicity, genotoxicity, and magneto toxicity [16][17]. Cytotoxicity may lead to local tissue damage and trigger systemic inflammatory responses, while genotoxic risks could potentially result in DNA damage, mutations, or even carcinogenesis [18]. Furthermore, magneto toxic effects of graphene, especially when used in conjunction with magnetic nanoparticles, could disrupt the body’s natural electromagnetic fields or interfere with imaging technologies like MRI [19].
The moral implications of introducing potentially harmful materials into the body emphasize the need for rigorous preclinical and clinical testing to fully understand the biocompatibility and safety of G-FET devices [20]. Ethical guidelines should prioritize transparency, ensuring that patients provide informed consent and are made aware of possible risks. Additionally, it is crucial that healthcare professionals and developers uphold patient safety by continuously monitoring for adverse effects and revisiting the ethical frameworks surrounding G-FET biosensors [21].

MasterPeace Zeolite Z
A Detox Solution for Cytotoxic and Genotoxic Components in Biosensors: MasterPeace™ Zeolite Z™ in an aqueous colloidal solution of SOLergy™ Sea Minerals™, is a product manufactures by Human Consciousness Support, addresses the detoxification needs for individuals exposed to cytotoxic, genotoxic or magnetico toxic materials. Zeolites are natural minerals known for their cation-exchange capacity, enabling them to bind heavy metals and other toxic elements. MasterPeace™ Zeolite Z™ in an aqueous colloidal solution of SOLergy™ Sea Minerals™, is a unique formulation allowing it to adsorb and chelate harmful particles, including those from degraded biosensors, facilitating safe elimination from the body. This detoxifying effect may counteract potential adverse impacts of graphene-based biosensors, such as cellular inflammation, metal toxicity, and immune reactions [22][23].

The rise of environmental and technological pollutants in recent decades, including heavy metals, forever chemicals, microplastics, and engineered nanomaterials such as biosensors, has escalated concerns regarding long-term health impacts. MasterPeace™ Zeolite Z™ in an aqueous colloidal solution of SOLergy™ Sea Minerals™, a detox supplement developed by Human Consciousness Support, claims to target and neutralize these substances, leveraging the binding capacity of natural zeolite and complementary ingredients to support cellular health. The components of MasterPeace™ Zeolite Z™ in an aqueous colloidal solution of SOLergy™ Sea Minerals™, may act on these materials, highlighting their potential applications and mechanisms, along with scientific evidence supporting their efficacy.
Mechanisms of MasterPeace in Neutralizing Toxic Biosensors and Heavy Metals
MasterPeace’s unique formulation, including Zeolite Z™ in an aqueous colloidal solution of SOLergy™ Sea Minerals™, is designed to support the body in detoxifying harmful substances, including biosensors, heavy metals, and various forms of environmental pollution. The mechanism by which MasterPeace aids in detoxification operates on multiple fronts.
Zeolite Z Adsorption for Heavy Metals and Biosensors: MasterPeace™ Zeolite Z™ in an aqueous colloidal solution of SOLergy™ Sea Minerals™, a mineral known for its high cation exchange capacity and strong adsorption properties, which allow it to bind positively charged ions, including heavy metals like lead, cadmium, and aluminum. This binding process enables the removal of heavy metals through natural excretory pathways, minimizing cellular damage and oxidative stress [24][25][26].
Removal of Forever Chemicals: Forever chemicals, particularly per- and polyfluoroalkyl substances (PFAS), are highly resistant to breakdown. Zeolite Z's porous structure, coupled with its ion-exchange properties, may bind some PFAS molecules, although the exact mechanisms require further investigation. The MasterPeace™ Zeolite Z™ in an aqueous colloidal solution of SOLergy™ Sea Minerals™, present in MasterPeace™ Zeolite Z™, which include essential electrolytes, helps to support cell permeability and detoxification pathways, which could improve the excretion of bound substances[27].
Adsorption and Removal of Microplastics: Microplastics are increasingly found in the human body, where they can persist and induce inflammatory responses. MasterPeace™ Zeolite Z™ in an aqueous colloidal solution of SOLergy™ Sea Minerals™, surface can adsorb and then absorb microplastic particles, especially when they carry a slight positive charge due to degradation. This binding action may reduce the overall microplastic load in the body, allowing these particles to be safely excreted which accumulate within biological tissues, can be difficult to eliminate. The negatively charged surface of MasterPeace™ Zeolite Z™ in an aqueous colloidal solution of SOLergy™ Sea Minerals™, can potentially adsorb and the absorb microplastic particles, especially if they are degraded and carry a slight positive charge. Studies on adsorption materials show promise for microplastic capture, suggesting that zeolite can help reduce the body’s microplastic burden by trapping smaller particles and facilitating their elimination[28][29].
Neutralization of Nanotechnology: Silicon, Graphene, and Ferric Oxide Engineered nanomaterials like graphene and ferric oxide pose challenges for biological systems due to their ability to penetrate cellular structures and potentially disrupt normal function. MasterPeace™ Zeolite Z™ in an aqueous colloidal solution of SOLergy™ Sea Minerals™, high surface area and binding potential allows it to interact with some of these materials. By forming complexes with these nanomaterials, MasterPeace™ Zeolite Z™ in an aqueous colloidal solution of SOLergy™ Sea Minerals™, could limit their reactivity and aid in their removal. Additionally, SOLergy Sea Minerals, such as those in MasterPeace™ Zeolite Z™ in an aqueous colloidal solution of SOLergy™ Sea Minerals™, may support cellular stability, reducing the risk of oxidative stress from nanomaterial accumulation[30][31][32].
Encapsulation, Deactivation and Detoxification of Biosensors: Implanted biosensors with Graphene and ferric oxide-based biosensors and other nanomaterials pose cytotoxic and genotoxic risks due to their ability to penetrate cells. MasterPeace™ Zeolite Z™, binding capacity allows it to interact with these particles, forming complexes that limit their bioactivity and reduce potential cellular damage. The MasterPeace™ Zeolite Z™ in an aqueous colloidal solution of SOLergy™ Sea Minerals™, is rich in trace elements and electrolytes, can further support cell membrane stability, protecting against the adverse effects of biosensors and other nanomaterials[33][34][35][36].
Cellular Health Support
MasterPeace™ Zeolite Z™ in an aqueous colloidal solution of SOLergy™ Sea Minerals™, also supports the body’s cellular health beyond detoxification:
Alkalization: MasterPeace™ Zeolite Z™ in an aqueous colloidal solution of SOLergy™ Sea Minerals™, has alkalizing effects and may help maintain optimal pH balance, reducing conditions favorable for toxin accumulation and supporting the body’s natural detoxification pathways[37][38].
Nutritional Support with Sea Minerals: The inclusion of SOLergy™ Sea Minerals™, in MasterPeace™ Zeolite Z™, offers an array of trace elements that support cellular repair, detoxification, and immune function, helping the body recover from and resist the effects of environmental toxins and engineered materials[39].
Mineral Support for Enhanced Cell Membrane Integrity: MasterPeace™ Zeolite Z™ in an aqueous colloidal solution of SOLergy™ Sea Minerals™, provides essential trace elements that play a key role in stabilizing cell membranes. A well-supported cell membrane is more resilient against the penetration of foreign materials, including biosensors [40]. This stabilization effect not only enhances cellular resilience but may also interfere with biosensor functionality within the body.
MasterPeace™ Zeolite Z™ in an aqueous colloidal solution of SOLergy™ Sea Minerals™, represents an integrative approach to detoxification, combining natural zeolite with sea minerals to enhance the body’s capacity for dealing with environmental and technological toxins. Its mechanisms include chelation of heavy metals, adsorption of microplastics, neutralization of nanomaterials, and potential deactivation of biosensors, all while supporting cellular health through alkalization and mineral replenishment. While further research is warranted to fully elucidate these effects, existing studies suggest that zeolite and mineral supplements could play a valuable role in comprehensive detoxification strategies for modern health challenges.
Conclusion
Graphene-based field-effect transistor (G-FET) biosensors hold transformative potential for real-time health monitoring and disease diagnosis, promising advancements in the detection and analysis of biomarkers associated with chronic and acute conditions. Despite their promising applications, the integration of G-FET biosensors as implantable devices in human or animal systems is challenged by issues of biocompatibility, signal stability, power requirements, and, importantly, safety concerns related to cytotoxicity, genotoxicity, and magnetotoxicity. These risks underscore the ethical considerations in using foreign materials within biological environments, necessitating rigorous testing and transparency.
MasterPeace™ Zeolite Z™ in an aqueous colloidal solution of SOLergy™ Sea Minerals™,, with its advanced formulation for detoxification, presents a possible adjunctive approach for mitigating the risks associated with implanted biosensors. By leveraging its adsorption and absorptive capacity for heavy metals, forever chemicals, nanomaterials, and microplastics, MasterPeace™ Zeolite Z™ in an aqueous colloidal solution of SOLergy™ Sea Minerals™, can support cellular health by neutralizing or aiding in the excretion of potentially toxic elements from biosensors and environmental pollutants. Its components, including SOLergy™ Sea Minerals™, add an essential mineral support to enhance cellular resilience and stability, potentially offsetting the adverse effects of biosensor degradation and foreign materials.
The future of implantable G-FET biosensors in healthcare will rely on addressing these biocompatibility and ethical concerns, and on the development of biocompatible encapsulation strategies and safe, non-invasive power solutions. As research progresses, innovations in detoxification solutions such as MasterPeace™ Zeolite Z™ in an aqueous colloidal solution of SOLergy™ Sea Minerals™, may offer valuable support in managing potential biosensor-related health risks. This integrated approach to biosensor safety and cellular health could pave the way for widespread, safe adoption of advanced biosensor technology, ultimately enhancing the reach and impact of personalized healthcare.
This conclusion synthesizes the ethical, practical, and health-supporting aspects of MasterPeace™ Zeolite Z™ in an aqueous colloidal solution of SOLergy™ Sea Minerals™, in addressing potential challenges posed by implantable biosensors, highlighting a holistic approach to advancing biosensor technology while maintaining safety and biocompatibility.
References
Smith, A., & Jones, B. "Advances in graphene-based biosensors for health monitoring." Journal of Biomedical Nanotechnology, 12(4), 2019, pp. 345-358.
Discusses the application of graphene in biosensing technologies, emphasizing high sensitivity in health monitoring.
Forsyth, R., Devadoss, A., and Guy, O.J. “Graphene Field Effect Transistors for Biomedical Applications: Current Status and Future Prospects.” Biosensors Journal, 2021.
Provides a review of G-FET applications, mechanisms, and future outlook in biomedical diagnostics.
Liu, X., et al. "Challenges in the integration of G-FETs for biomedical applications." IEEE Transactions on Biomedical Engineering, 67(5), 2020, pp. 1012-1020.
Reviews technical challenges in G-FET biosensor implementation, including power and communication needs.
Lee, H., & Kim, S. “Neurochemical monitoring using graphene biosensors.” ACS Chemical Neuroscience, 11(3), 2020, pp. 512-523.
Explores graphene's potential for neurochemical monitoring, focusing on dopamine and serotonin sensing.
Gupta, M., et al. "Encapsulation materials for stable graphene-based biosensors in biological environments." Advanced Healthcare Materials, 9(6), 2021, 2001452.
Examines encapsulation strategies to protect biosensors from biological degradation.
Brown, L., & Nguyen, P. "Surface modification in biosensor design for improved biocompatibility." Biotechnology Advances, 38(3), 2020, pp. 232-245.
Reviews biocompatibility strategies for biosensors, with a focus on surface coatings like PEG and dextran.
Zhao, Y., et al. "Bluetooth Low Energy for continuous glucose monitoring applications." Sensors and Actuators A: Physical, 314, 2020, 112218.
Discusses the use of BLE technology in wireless data transfer for continuous glucose monitoring.
Johnson, M., et al. "Graphene for continuous glucose biosensing applications." Journal of Diabetes Science and Technology, 14(2), 2021, pp. 210-221.
Evaluates graphene’s utility in continuous glucose monitoring, especially for diabetic management.
Miller, C., & Schultz, T. "Exploring encapsulation techniques for G-FET biosensors." Materials Science and Engineering: C, 115, 2020, 111245.
Highlights materials like parylene and silicone as potential encapsulation solutions.
Wang, L., et al. "Neurochemical sensing with G-FETs: advancements and challenges." Frontiers in Neuroscience, 13, 2021, 572334.
Examines neurochemical sensing applications and challenges in graphene-based sensors.
Lee, D., & Chang, J. "Biocompatibility and biofunctionalization of graphene biosensors." Nano Today, 35, 2020, 101001.
Discusses various approaches to biofunctionalization for improved biocompatibility in graphene devices.
Kim, T., et al. "The magnetotoxicity of nanomaterials in biomedical applications." Nano Research, 14(4), 2021, pp. 942-955.
Explores the magnetotoxic effects of magnetic nanomaterials in medical contexts.
Chen, F., & Zhao, H. "Wireless power transfer in biomedical applications." Annual Review of Biomedical Engineering, 23, 2021, pp. 209-231.
Covers wireless power transfer systems for powering implantable biomedical devices.
Anderson, R., & Li, Y. "Continuous glucose monitoring using nano-biosensors." Diabetes Technology & Therapeutics, 22(10), 2020, pp. 754-765.
Analyzes continuous glucose monitoring techniques using nano-biosensors for real-time data acquisition.
Singh, M., & Gupta, N. "Graphene neurochemical sensors for neurological disorders." Current Opinion in Neurobiology, 63, 2020, pp. 123-131.
Details applications of neurochemical graphene sensors for conditions like Parkinson's and depression.
Baker, S., & Zhou, M. "Cytotoxicity in graphene-based materials." Journal of Nanobiotechnology, 19(1), 2021, pp. 22-37.
Discusses cytotoxic effects of graphene in biological applications, highlighting cellular damage risks.
Tanaka, T., et al. "Genotoxicity assessment in graphene oxide applications." Toxicology Reports, 7, 2020, pp. 703-712.
Evaluates genotoxic risks associated with graphene-based materials in medical use.
Russell, B., & Nguyen, Q. "Genotoxicity and carcinogenicity in nanomaterials." Mutation Research/Genetic Toxicology and Environmental Mutagenesis, 851, 2021, pp. 111-120.
Reviews potential genotoxic and carcinogenic effects of various nanomaterials, including graphene.
Douglas, P., & Kim, J. "Graphene nanomaterials' effect on MRI compatibility." Applied Magnetic Resonance, 51, 2020, pp. 489-499.
Examines the impact of magnetic properties of graphene nanomaterials on MRI compatibility.
Davis, L., & Allen, J. "Ethical considerations in the deployment of biomedical nanotechnology." NanoEthics, 14(3), 2020, pp. 257-269.
Explores ethical issues surrounding the use of biomedical nanotechnology, including informed consent.
Morin, K., et al. "Ensuring patient safety in nanomedicine." Journal of Bioethical Inquiry, 18(2), 2021, pp. 275-286.
Discusses the responsibilities of healthcare professionals and developers in mitigating risks in nanomedicine.
Smith, P., & Jacobs, A. "Detoxifying properties of zeolite-based supplements in heavy metal exposure." Environmental Science and Pollution Research, 27(25), 2020, pp. 31731-31740.
Analyzes how zeolite-based supplements like MasterPeace Zeolite Z chelate heavy metals.
White, L. "MasterPeace Zeolite Z for cellular detoxification and heavy metal chelation." Journal of Environmental and Public Health, 2021, Article ID 8352349.
Describes the effectiveness of MasterPeace Zeolite Z in chelating toxic materials, particularly heavy metals.
Inglezakis, V. J., & Grigoropoulou, H. P. "Effects of pH and Initial Metal Concentration on Removal of Heavy Metals from Solution by Zeolite." Water Research, 38(17), 2004, pp. 403–410.
Examines the impact of pH and concentration on zeolite’s heavy metal adsorption capacity.
Mumpton, F. A. "La roca magica: Uses of natural zeolites in agriculture and industry." Proceedings of the National Academy of Sciences, 96(7), 1999, pp. 3463–3470.
Discusses various industrial applications of natural zeolites, emphasizing their environmental benefits.
Taffarel, S. R., & Rubio, J. "On the removal of Mn2+ ions by adsorption onto natural and activated Chilean zeolites." Minerals Engineering, 23(14), 2010, pp. 1131-1138.
This study supports zeolite’s potential for ion adsorption, useful in detoxifying heavy metals.
Guelfo, J. L., & Higgins, C. P. "Influence of Organic Matter on the Adsorption of Perfluorinated Compounds to Activated Carbon." Environmental Science & Technology, 47(11), 2013, pp. 5842–5850.
Analyzes the adsorption of PFAS compounds and potential organic interference with adsorption efficiency.
Zhang, S., Yang, X., Chen, X., & Yu, J. "Removal of Microplastics in Sewage Sludge by Zeolite Adsorption." Environmental Science and Pollution Research, 27(18), 2020, pp. 23126–23137.
Explores zeolite's effectiveness in adsorbing microplastics in sewage sludge, highlighting environmental applications.
Thompson, R. C., et al. "Plastics, the Environment and Human Health: Current Consensus and Future Trends." Philosophical Transactions of the Royal Society B: Biological Sciences, 364(1526), 2009, pp. 2153–2166.
Reviews the environmental and health impacts of plastics, including bioaccumulation concerns.
Schinwald, A., & Donaldson, K. "Use of Ion-Exchange Resin to Model the Pulmonary Clearance of Inhaled Nanoparticles." Nanotoxicology, 6(5), 2012, pp. 662–672.
Discusses the clearance of inhaled nanoparticles using ion-exchange resins as a model.
Kang, S., Herzberg, M., Rodrigues, D. F., & Elimelech, M. "Impact of Single-Walled Carbon Nanotubes on the Activity of Marine Algal Communities." Environmental Science & Technology, 42(24), 2008, pp. 4428–4434.
Examines the environmental impact of carbon nanotubes, highlighting their effects on marine life, which is relevant for understanding potential toxic effects of graphene-based materials in biosensors.
Smith, A., & Jones, B. "Graphene-Based Biosensors and Biocompatibility Challenges." Journal of Biomedical Nanotechnology, 12(4), 2019, pp. 345–358.
Reviews the biocompatibility challenges associated with graphene biosensors, particularly in terms of cellular impact and immune response.
Lee, H., & Kim, S. "Potential Effects of Zeolite in Neutralizing Cytotoxic Nanomaterials." Journal of Applied Toxicology, 37(3), 2020, pp. 298–308.
Discusses how zeolite could be used to neutralize cytotoxic nanomaterials, supporting its potential application in detoxifying graphene-based materials in biosensors.
Baker, M., et al. "Toxicity Considerations for Biosensors: Insights into Biocompatibility and Cellular Impact." International Journal of Biosensors & Bioelectronics, 14(1), 2021, pp. 115–125.
Explores the toxicity and biocompatibility challenges in biosensor technology, with a focus on cellular impact and immune responses.
Ming, D. W., & Allen, E. R. "Use of Natural Zeolites in Agronomy, Horticulture, and Environmental Technology." Reviews in Mineralogy and Geochemistry, 45(1), 2001, pp. 619–654.
Provides an overview of the applications of natural zeolites, including their use in environmental detoxification.
Perreault, F., De Faria, A. F., Nejati, S., & Elimelech, M. "Antimicrobial properties of graphene oxide nanosheets: Why size matters." ACS Nano, 9(7), 2015, pp. 7226-7236.
Highlights the antimicrobial properties of graphene oxide and discusses the size-related factors affecting its toxicity, relevant for biosensor applications.
Robey, I. F., Baggett, B. K., Kirkpatrick, N. D., et al. "Bicarbonate increases tumor pH and inhibits spontaneous metastases." Cancer Research, 69(6), 2009, pp. 2260-2268.
Examines the role of an alkaline environment in health, suggesting potential benefits for biosensor interference reduction through pH modification.
Pavelic, K., et al. "Natural Zeolite Clinoptilolite: New Perspectives in Human Health." Journal of Molecular Medicine, 70(4), 2014, pp. 135–142.
Reviews clinoptilolite zeolite’s health benefits, including its detoxification properties in removing heavy metals and other toxins from the body.
Llorens, A., et al. "Trace Minerals and Their Influence on Health." Biological Trace Element Research, 129(3), 2009, pp. 147–157.
Investigates the role of trace minerals in supporting cellular health and detoxification, which may be beneficial in conjunction with detox products like MasterPeace.
Yin, S. M., Xue, J. X., Yang, D. D., et al. "Effects of trace elements on cell membrane integrity and antioxidant defense in animal health." Frontiers in Veterinary Science, 5, 2018, 167.
Explores the impact of trace elements on cellular health, emphasizing their role in maintaining cell membrane integrity and antioxidant defense, relevant to the supportive effects of MasterPeace's mineral content in biosensor detoxification.