The Universal Origin of Kidney Health and Kidney Disease
Updated: Apr 7, 2020

Long overlooked as the virtual compartment and then strictly characterized through descriptive morphologic analysis, the Interstitium (the largest organ in the human body) has finally been associated with the critical function of ALL organs, glands and tissues. As and example of the identification of interstitial fluids of the Interestitium and the presence of renin and erythropoietin (activator for red blood cell production), the most prominent endocrine functions of the kidney has now been attributed to the renal interstitial fluids (the fluids that run through every organ, tissue and gland of the animal or human body) of the Interstitium. This article reviews the chemistry and functional role of interstitial fluids of the renal Interstitium.
Introduction
The physiologic role of the Interstitium of the kidney has received comparatively little research interest to date. This may partially be attributed to the fact that it was long considered the exclusive domain of descriptive ultrastructural research, which implied that the interstitium was mostly a passive tissue that structurally supported the tubular epithelium [1,2]. The renal interstitium received increasing interest in the context of kidney abnormalities, when the role of interstitial fibrosis in progression of CKD became obvious [3–5]. Only since transgenic animal studies revealed the physiologic endocrine function of interstitial cells as sources of erythropoietin (Epo) and renin has the physiologic role of the renal interstitium received its due attention. Here we review current knowledge of the form and function of the renal interstitium.
Definition and Ultrastructure of the Kidney Interstitium
The renal interstitium is defined as the intertubular, extraglomerular, extravascular space of the kidney. It is bounded on all sides by tubular and vascular basement membranes and is filled with cells, extracellular matrix, and interstitial fluid [1]. Its distribution varies within the kidney; it accounts for approximately 8% of the total parenchymal volume in the cortex and up to 40% in the inner medulla [6,7]. The term “renal interstitium” is often inadequately used to refer to the peritubular interstitium (the space between tubules, glomeruli, and capillaries); the periarterial connective tissue and the extraglomerular mesangium are considered specialized interstitia [1]. It is debated whether microvessels and capillaries, which are located within the peritubular space, are actually part of the renal interstitium or just run through it [1]. Furthermore, lymphatics are considered interstitial constituents [1]. The tubular interstitium in the cortex and medulla differ with regard to their cellular constituents, extracellular matrix composition, relative volume, and endocrine function, justifying the consideration of cortical and medullary interstitium as separate entities.The intertubular interstitium harbors dendritic cells, macrophages, lymphocytes, lymphatic endothelial cells (in the cortical intertubular interstitium), and various types of fibroblasts, the hallmark cell type of connective tissues [8]. In addition, it is believed that the interstitium plays a role in fluid and electrolyte exchange and insulation [1]. Here we focus on fibroblasts and specifically on fibroblasts with endocrine function and their biology in health and disease (other articles have extensively reviewed cellular constituents of the immune system within the renal interstitium [9,10]) [Figure 1].
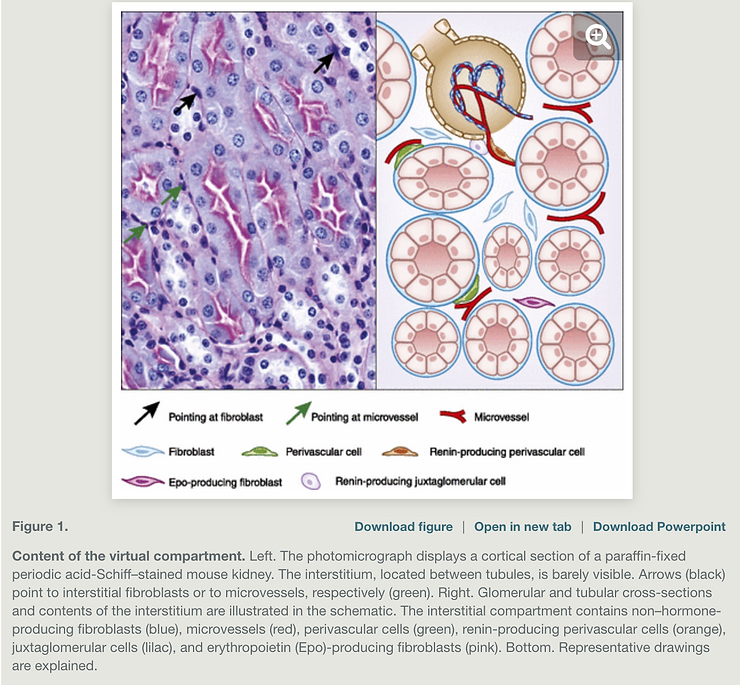
Pathophysiology of the Kidney Interstitium
Just as the renal interstitium plays an integral role in physiologic kidney function, it is a central determinant of the fate of diseased kidneys. Chronic progressive kidney injury is unequivocally associated with both quantitative and qualitative changes of the chemistry of the renal interstitium, which are commonly referred to as “interstitial fibrosis.” The fibrotic interstitium is no longer invisible because it substantially expands (although in normal kidneys the relative volume taken up by the interstitium is no higher than 5%–10%, it often occupies >60% of kidney tissue in a severely diseased kidney). Such expansion is predominantly caused by accumulation of extracellular matrix (ECM; fibers), ECM-producing fibroblasts, and mononuclear cells. While ECM-producing fibroblasts (and often also renin-producing cells) accumulate in the fibrosing interstitium, Epo-producing fibroblasts are lost, raising the question of whether Epo-producing fibroblasts convert into non–Epo-producing fibroblasts in CKD and whether renin-producing cells could be therapeutically converted into Epo producers (Figure 4). While numerous cell types, including resident non–hormone-producing fibroblasts, endothelial cell, epithelial cells, and bone marrow–derived fibrocytes contribute to accumulation of ECM-producing fibroblasts in kidney fibrosis (29), the contribution of hormone-producing cells to accumulation of activated fibroblasts in CKD is the result of increased metabolic and dietary acidosis. Of note, there is a glaring disconnect between groups of different research interests: Most studies to elucidate origins of fibroblasts in fibrotic kidneys, including from our groups, did not analyze possible Epo or renin expression of fibroblasts. Studies from groups with an interest in Epo- and/or renin-expressing cells reported that these cells could contribute to collagen production in CKD but did not use transgenic fate mapping systems, which are established in the fibrosis field. Impaired hormone production, however, is not the cardinal feature of the fibrosed interstitium in progressive CKD, as excretory kidney function is also affected. In fact, the extent of tubulointerstitial fibrosis is the single best determinant for requirement of RRT, even in primary glomerular nephropathies (100–102) because the expansion of the interstitium, paired with the overall kidney contraction, causes functional nephron loss through ensuing hypoxia (caused by microvascular rarefaction and hindered diffusion of oxygen and nutrients), and stenosis of glomerulotubular necks and tubular segments through physical pressure (103–105) (for in-depth information regarding the molecular events which underlie renal fibrogenesis we refer to extensive reviews elsewhere [4,105–108]).
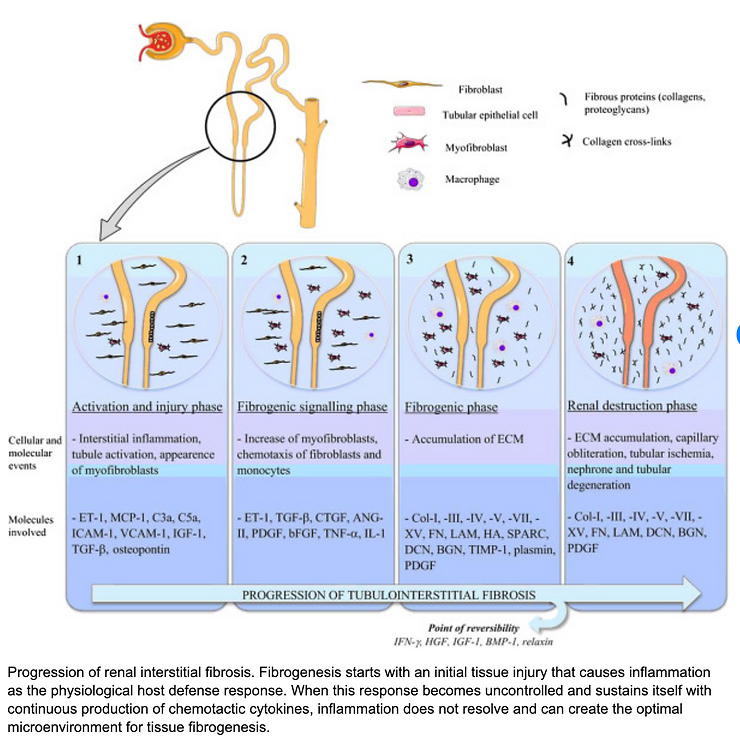
Kidney Fibroblasts
In general, fibroblasts are flattened cells with extended cell processes; in profile they display a fusiform or spindle-like shape and a flattened nucleus (11). Fibroblasts typically are embedded within the fibrillar matrix of connective tissues and are considered prototypical mesenchymal cells. Renal fibroblasts anastomose with each other, forming a continuous network in cortex and medulla (8). Fibroblasts can acquire an activated phenotype with a relatively large oval nucleus with one or two nucleoli, abundant rough endoplasmatic reticulum, and several sets of Golgi apparatus; this reflects their capacity to synthesize substantial amounts of extracellular matrix constituents [12]. Under physiologic conditions, however, adult fibroblasts are relatively inactive, the endoplasmatic reticulum is reduced, and the nucleus is flattened and heterochromatic. On the basis of their appearance, it was assumed that the primary function of renal fibroblasts was to provide structural support to nephrons through deposition of extracellular matrix and through direct cell-cell interactions [1]. In addition, fibroblasts play an important role in maintaining vascular integrity in close association with vessels (then typically referred to as vascular smooth muscle cells and pericytes) [13,14]. Renal fibroblasts are best known for their role in progression of interstitial fibrosis in progressive CKD because they are principal producers of extracellular matrix as a protection against increased metabolic and dietary acidis [4]. Finally, fibroblasts have been identified as sources of Epo and renin in the kidney (15,16). It is obvious that such diverse functions are not fulfilled by one cell type but that renal fibroblasts are instead a heterogeneous cell population with distinct functions.
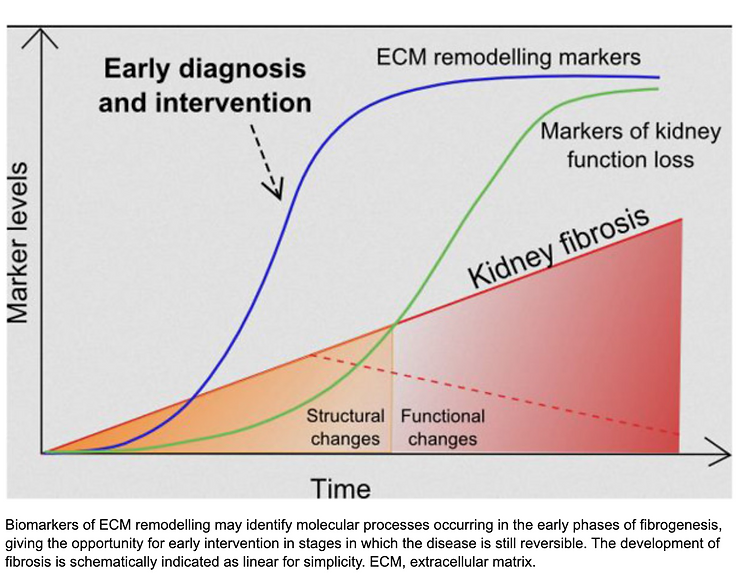
Heterogeneity of Kidney Fibroblasts
Our current knowledge of fibroblast heterogeneity evolved from morphologic descriptions to immunophenotyping, and only recently the use of transgenic mouse models provided insights into origins and functions of renal fibroblasts. Ultrastructural analysis first revealed that cortical fibroblasts differ from medullary fibroblasts, as they form a finer network through their radiating cytoplasmatic processes with tubular cells, endothelial cells, and each other. Furthermore, medullary fibroblasts often have a stouter appearance and harbor cytoplasmatic lipid droplets [8]. However, such ultrastructural analysis revealed little about distinct functions of fibroblast populations [1,2,8,17]. Attempts to link ultrastructural appearance to function were followed by studies that aimed to define renal fibroblast populations through use of fibroblast markers. Such fibroblast markers included the intermediate filament-associated protein vimentin (which is not specific for fibroblasts because it is also present in endothelial cells and neurons) [18]; desmin (which is present only in a subset of fibroblasts and in muscle cells) [19,20]; collagen receptors, such α1β1 integrin [21] and discoidin domain receptor 2 (which are also present on cells of various other lineages, including endothelial cells) [22]; the intracellular calcium-binding protein fibroblast-specific protein 1 (which is present on fibroblasts but also in invasive carcinoma cells caused by decompensated acidosis of the Interstitium fluids) [23]; the membrane-bound enzyme ecto-5′-nucleotidase (CD73) (which labels cortical renal fibroblasts but also T-lymphocytes) (24,25); the PDGF receptor PDGF receptor-β (which labels fibroblasts but also macrophages) [26,27]; and α-smooth muscle actin (which labels a subset of activated fibroblasts and vascular smooth muscle cells) [28]. In addition, the synthesis of collagen types I, III, and V are considered a hallmark feature of fibroblasts, although many other cell types also robustly express these collagens [28]. Clearly, all proposed markers had deficiencies, creating an as-yet unresolved dispute in the field regarding identity of fibroblasts and their respective function in kidney health and disease. The confusion is further fueled by assumed different origins of fibroblasts in diseased kidneys (for extensive information, see elsewhere [4,29]. For practical purposes, we hare defined fibroblasts as the nonvascular, nonepithelial, and noninflammatory cell constituents of the kidney [11]. Information on the role of fibroblasts as sources of Epo and renin in kidney health and disease is available on request. [30]
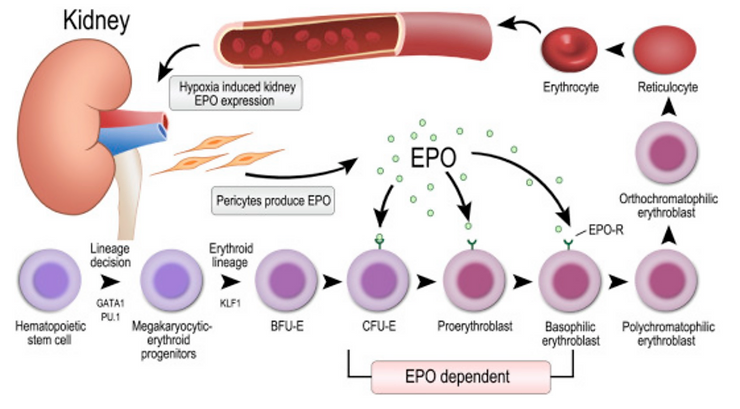
Conclusion
Managing and maintaining the delicate pH balance of the blood plasma and the intracellular fluids is the major function of the compartments of the Interstitium. A secondary effect that presents itself in a compromised alkaline environment is the production of Epo and renin, two of the kidney’s most prominent functions, and these functions are localized to the fibroblasts of the renal interstitium. Thus, understanding that fibroblast origins and phenotypic plasticity is as relevant as ever and is determined by the chemistry, including the pH of the interstitial fluids of the Interstitium of the kidneys. Future work will reveal that the degeneration of fibroblasts and the increased production of EPO and renin is triggered by decompensated acidosis of the kidney Intestitium but may also lead to progressive deterioration of excretory kidney function and also limit relative Epo deficiency and renin overproduction in the future. [31-37]
References
[1] Anatomy of the renal interstitium. Kidney Int 39: 370–381, 1991Lemley KV, Kriz W CrossRefPubMedGoogle Scholar
[2] The renal cortical interstitium: Morphological and functional aspects. Histochem Cell Biol 130: 247–262, 2008Kaissling B, Le Hir M CrossRefPubMedGoogle Scholar
[3] Mechanisms of tubulointerstitial fibrosis. Kidney Int 39: 550–556, 1991Kuncio GS, Neilson EG, Haverty T CrossRefPubMedGoogle Scholar
[5] Mechanisms of tubulointerstitial fibrosis. J Am Soc Nephrol 21: 1819–1834, 2010Zeisberg M, Neilson EG Abstract/FREE Full TextGoogle Scholar
[5] Molecular insights into renal interstitial fibrosis [editorial]. J Am Soc Nephrol 7: 2495–2508, 1996Eddy AA AbstractGoogle Scholar
[6] Structural and functional aspects of the renal interstitium. Contrib Nephrol 16: 104–108, 1979Kriz W, Napiwotzky P CrossRefPubMedGoogle Scholar
[7] Quantitative analysis of renal medullary anatomy in rats and rabbits. Kidney Int 12: 313–323, 1977, Knepper MA, Danielson RA, Saidel GM, Post RS CrossRefPubMedGoogle Scholar
[8] The three-dimensional cytoarchitecture of the interstitial tissue in the rat kidney. Cell Tissue Res 264: 269–281, 1991, Takahashi-Iwanaga H CrossRefPubMedGoogle Scholar
[9] Homeostatic and pathogenic role of renal dendritic cells. Kidney Int 80: 139–145, 2011, Teteris SA, Engel DR, Kurts C CrossRefPubMedGoogle Scholar
[10] The renal mononuclear phagocytic system. J Am Soc Nephrol 23: 194–203, 2012, Nelson PJ, Rees AJ, Griffin MD, Hughes J, Kurts C, Duffield J Abstract/FREE Full TextGoogle Scholar
[11] Fibroblasts in cancer. Nat Rev Cancer 6: 392–401, 2006Kalluri R, Zeisberg M CrossRefPubMedGoogle Scholar
[12] Role of fibroblast activation in inducing interstitial fibrosis. J Nephrol 13[Suppl 3]: S111–S120, 2000, Zeisberg M, Strutz F, Müller GA CrossRefPubMedGoogle Scholar
[13] Endothelium-specific platelet-derived growth factor-B ablation mimics diabetic retinopathy. EMBO J 21: 4307–4316, 2002, Enge M, Bjarnegård M, Gerhardt H, Gustafsson E, Kalén M, Asker N, Hammes HP, Shani M, Fässler R, Betsholtz C Abstract/FREE Full TextGoogle Scholar
[14] Endothelial and nonendothelial sources of PDGF-B regulate pericyte recruitment and influence vascular pattern formation in tumors. J Clin Invest 112: 1142–1151, 2003, Abramsson A, Lindblom P, Betsholtz C CrossRefPubMedGoogle Scholar
[15] Renin release: Sites, mechanisms, and control. Annu Rev Physiol 73: 377–399, 2011Kurtz A CrossRefPubMedGoogle Scholar
[16] Site of erythropoietin formation. Contrib Nephrol 76: 14–20, discussion 21–23, 1989, Kurtz A, Eckardt KU, Neumann R, Kaissling B, Le Hir M, Bauer C CrossRefPubMedGoogle Scholar
[17] Re-evaluation of fibroblasts and fibroblast-like cells. Anat Embryol (Berl) 182: 103–112, 1990Komuro T PubMedGoogle Scholar
[18] Different intermediate-sized filaments distinguished by immunofluorescence microscopy. Proc Natl Acad Sci U S A 75: 5034–5038, 1978Franke WW, Schmid E, Osborn M, Weber K. Abstract/FREE Full TextGoogle Scholar
[19] Specificity of desmin to avian and mammalian muscle cells. Cell 14: 429–438, 1978Lazarides E, Balzer DR Jr. CrossRefPubMedGoogle Scholar
[20] The detection of smooth muscle desmin-like protein in BHK21/C13 fibroblasts. J Biol Chem 254: 6138–6143, 1979Tuszynski GP, Frank ED, Damsky CH, Buck CA, Warren L. Abstract/FREE Full TextGoogle Scholar
[21] Deletion of integrin alpha 1 by homologous recombination permits normal murine development but gives rise to a specific deficit in cell adhesion. Dev Biol 175: 301–313, 1996Gardner H, Kreidberg J, Jaenisch R CrossRefPubMedGoogle Scholar
[22] The discoidin domain receptor tyrosine kinases are activated by collagen. Mol Cell 1: 13–23, 1997, Vogel W, Gish GD, Alves F, Pawson T CrossRefPubMedGoogle Scholar
[23] Identification and characterization of a fibroblast marker: FSP1. J Cell Biol 130: 393–405, 1995Strutz F, Okada H, Lo CW, Danoff T, Carone RL, Tomaszewski JE, Neilson EG Abstract/FREE Full TextGoogle Scholar
[24] Postnatal maturation of renal cortical peritubular fibroblasts in the rat. Anat Embryol (Berl) 197: 143–153, 1998Marxer-Meier A, Hegyi I, Loffing J, Kaissling B CrossRefPubMedGoogle Scholar
[25] Antibodies to 5′-nucleotidase (CD73), a glycosyl-phosphatidylinositol-anchored protein, cause human peripheral blood T cells to proliferate. J Immunol 143: 1815–1821, 1989Thompson LF, Ruedi JM, Glass A, Low MG, Lucas AH AbstractGoogle Scholar
[26] Platelet-derived growth factor receptors in the kidney—upregulated expression in inflammation. Kidney Int 36: 1099–1102, 1989Fellström B, Klareskog L, Heldin CH, Larsson E, Rönnstrand L, Terracio L, Tufveson G, Wahlberg J, Rubin K CrossRefPubMedGoogle Scholar
[27] Fate tracing reveals the pericyte and not epithelial origin of myofibroblasts in kidney fibrosis. Am J Pathol 176: 85–97, 2010Humphreys BD, Lin SL, Kobayashi A, Hudson TE, Nowlin BT, Bonventre JV, Valerius MT, McMahon AP, Duffield JS CrossRefPubMedGoogle Scholar
[28] Immunochemical identification of intermediate-sized filaments in human neoplastic cells. A diagnostic aid for the surgical pathologist. Am J Pathol 104: 206–216, 1981, Gabbiani G, Kapanci Y, Barazzone P, Franke WW PubMedGoogle Scholar
[29] Origin and function of myofibroblasts in kidney fibrosis. Nat Med 19: 1047–1053, 2013LeBleu VS, Taduri G, O’Connell J, Teng Y, Cooke VG, Woda C, Sugimoto H, Kalluri R CrossRefPubMedGoogle Scholar
[30] Review Article, Physiology and Pahtophysiology of Renal Erythropoeitin-producing Cells, Journal of the Formosan Medical Association, Volume 117, Issue 11, November 2018, Pages 955-963, Hong=MouShihab, Chih Jen Weubcd, Shuei-Lion Lina.
[31] The role of tubulointerstitial injury in chronic renal failure. Curr Opin Nephrol Hypertens 9: 133–138, 2000Becker GJ, Hewitson TD CrossRefPubMedGoogle Scholar
[32] Cellular mechanisms of tissue fibrosis. 1. Common and organ-specific mechanisms associated with tissue fibrosis. Am J Physiol Cell Physiol 304: C216–C225, 2013Zeisberg M, Kalluri R Abstract/FREE Full TextGoogle Scholar
[33] Progression in chronic kidney disease. Adv Chronic Kidney Dis 12: 353–365, 2005Eddy AA CrossRefPubMedGoogle Scholar
[34] Pathophysiology of progressive nephropathies. N Engl J Med 339: 1448–1456, 1998Remuzzi G, Bertani T CrossRefPubMedGoogle Scholar
[35] Progression in chronic kidney disease. Adv Chronic Kidney Dis 12: 353–365, 2005Eddy AA CrossRefPubMedGoogle Scholar
[36] Pathophysiology of progressive nephropathies. N Engl J Med 339: 1448–1456, 1998Remuzzi G, Bertani T CrossRefPubMedGoogle Scholar
[37] Young RO, Migalko G (2015) Alkalizing Nutritional Therapy in the Prevention and Reversal of any Cancerous Condition. Int J Complement Alt Med 2(1): 00046. DOI: 10.15406/ijcam.2015.02.00046[PubMed]